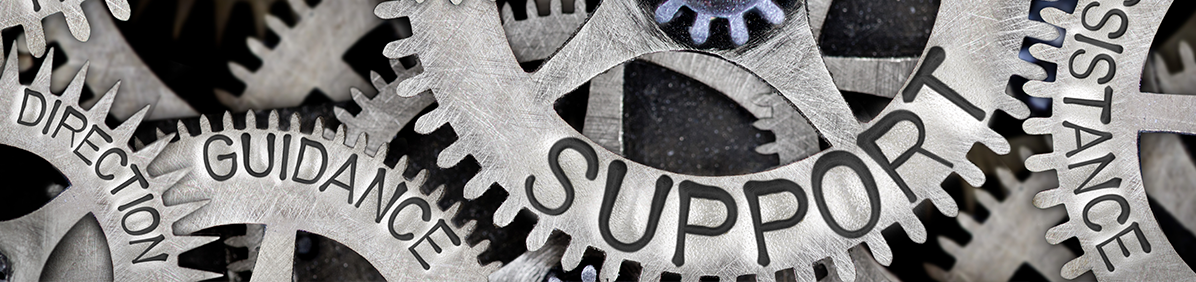
new wave of Wi-Fi is here! The IEEE 802.11ax Enhancements for High Efficiency Wireless (HEW) LAN standard1 has far reaching implications with respect to cabling infrastructure design. Users can expect their current wireless speeds to appreciably increase by switching to Wi-Fi 6 gear with greater than 5 Gb/s data rate capability. Plus, 1024-QAM modulation, 160 MHz channel bandwidth, and a maximum of eight spatial streams can theoretically deliver 9.61 Gb/s in the future! Now more than ever, the specification of high performance cabling supporting access layer switches and uplink connections is critical to achieving multi-Gigabit throughput and fully supporting the capacity of next generation wireless access points.
Wi-Fi 6 delivers increased speed and capacity and more efficiently supports the increasing density of devices, while lowering latency and enhancing battery life. Growth for Wi-Fi 6 solutions will be explosive; with IHS Markit™ estimating IEEE 802.11ax-enabled device shipments to reach 193 million units in 20212. Specifying products that are part of the Wi-Fi Alliance® Wi-Fi CERTIFIED 6™ program is a recommended way to ensure that wireless products meet IEEE 802.11ax standards for interoperability and security.
Key cabling design strategies to ensure that structured cabling uplinks are ready to support the Wi-Fi 5 and Wi-Fi 6 wireless LAN devices addressed in this paper include:
Siemon’s shielded category 6A and category 7A cables are qualified for mechanical reliability up to 75°C (167°F), which enables support of the Type 2 PoE application over the entire operating temperature range of -20°C to 60°C (-4°F to 140°F).
Shielded systems are more thermally stable and support longer channel lengths when deployed in high temperature environments and larger number of shielded cables may be bundled without concern for excessive heat build-up within the bundle.
Wi-Fi 6 has four times faster average throughput in dense deployment environments compared to Wi-Fi 5. This enhanced throughput is facilitated by an evolution of existing and proven Wi-Fi-5 communication algorithms. Like Wi-Fi 5, Wi-Fi 6 wireless transmission utilizes the techniques of beamforming to concentrate signals and transmitting over multiple send and receive antennas to improve communication and minimize interference (often referred to as multiple input, multiple output or MIMO). The signal associated with one transmit and one receive antenna is called a spatial stream and the ability to support multiple spatial streams is a feature of Wi-Fi 4, Wi-Fi 5, and Wi-Fi 6. Higher order modulation, an orthogonal frequency-division multiple access (OFDMA) signal scheme, which allows bandwidth to be divided according to the needs of the client, and synchronized uplink transmission are the key technology enablers that support faster Wi-Fi 6 transmission rates while ensuring backward compatibility with older Wi-Fi technology.
Quadrature amplitude modulation (QAM) is an analog and digital modulation scheme that is used extensively for digital telecommunications systems. Using this scheme, a four quadrant arrangement or “constellation” of symbol points is established with each point representing a short string of bits (e.g. 0’s or 1’s). Sinusoidal carrier waves that are phase shifted by 90 degrees are modulated using amplitude-shift keying (ASK) digital modulation or amplitude modulation (AM) analog modulation schemes and are used to transmit the constellation symbols. Figure 1 depicts a rudimentary example of a 16-QAM constellation for demonstration purposes. Note that there are four points in each quadrant of the 16-QAM constellation and each point equates to four information bits, ranging from 0000 to 1111. By comparison, the Wi-Fi 4 64-QAM scheme carries 6 bits of information per constellation point, the Wi-Fi 5 256 QAM scheme carries 8 bits of information per constellation point, and the Wi-Fi 6 1024-QAM carries an amazing 10 bits of information per constellation point.
Unlike Wi-Fi 5 devices that transmit exclusively in the 5 GHz spectrum, Wi-Fi 6 devices benefit from operation in both the 2.4 GHz and 5 GHz bands. This balances higher transmission rates achievable in the 5 GHz spectrum where there are more available non-overlapping radio channels, fewer devices operating, and less potential for interference with opportunities to transmit in the 2.4 GHz band where superior propagation characteristics (e.g., range and the ability to pass through building materials) may be better suited for IoT (Internet of Things) applications. Designing a flexible cabling infrastructure that can accommodate the addition of future WAPs and enable rapid reconfiguration of coverage areas can save headaches later. Figure 2 depicts a recommended zone cabling approach utilizing enclosures that house service concentration points (SCPs) with spare port capacity to facilitate connections to service outlets (SOs) that are positioned in a grid pattern. In addition, because most WAPs are located in the ceiling or in plenum spaces where higher temperatures are likely to be encountered, the use of solid conductor cords, which exhibit better thermal stability and lower insertion loss than stranded conductor cords3, are recommended for all equipment connections in high temperature environments. Refer to ANSI/BICSI 0074 and TIA TSB-162-A5 for additional design and installation guidelines describing a grid-based cabling approach that maximizes WAP placement and reconfiguration flexibility.
Because of the variables of channel bandwidth, number of spatial streams, and multi-user signaling mechanisms,
Wi-Fi 5 and Wi-Fi 6 implementations are highly configurable. In general, the lower end of the throughput range is targeted for small handheld devices with limited battery capacity such as smart phones, the middle of the throughput range is targeted towards laptops, and the highest end of the throughput range is targeted at specialized and outdoor applications where there is less device density compared with indoors. Figure 4 provides maximum theoretical speeds for currently available first and second wave Wi-Fi 5 and emerging Wi-Fi 6 implementations with their target end devices.
Possible future Wi-Fi 6 implementations are also shown, but these implementations may not be available for years, if at all. While this may seem surprising, consider that there are no 4-stream implementations of Wi-Fi 4 and manufacturers are bypassing 8-stream Wi-Fi 5 implementations in favor of Wi-Fi 6 products. Second wave Wi-Fi 6 devices will make use of multi-antenna beamforming, more efficient spatial utilization, and other strategies to improve throughput and long-range operation. The bottom line is that end-users can reasonably expect four times faster performance in dense environments and similar or slightly improved power consumption upon making the upgrade to Wi-Fi 6 gear.
When comparing Wi-Fi capabilities, it’s important to keep in mind that the maximum realizable data rate is impacted by the number of clients, protocol overhead, and the spatial distribution of end-user devices from the access point. In some cases, Wi-Fi 5 wireless data transfer rates are fast enough to saturate a 1000BASE-T copper balanced twisted-pair cabling link provided between the router and the server6. Proliferation of Wi-Fi 6 clients will certainly result in data rates regularly exceeding 1 Gb/s. 2.5GBASE-T and 5GBASE-T switches can reduce or eliminate the concern that a standard Gigabit Ethernet wired uplink ports will be a bottleneck to data transmission. However, it’s important to keep in mind that only class EA/category 6A and higher rated cabling is guaranteed to support 2.5/5GBASE-T over all installation environments and channel topologies up to 100 m.
The ability of Wi-Fi 6 to realize greater than 5 Gb/s wireless data rate capability has serious implications related to wired media selection for router to server and other uplink connections. Wi-Fi 6 access points connect into the Ethernet network with two ports rather than the traditional one port. Across all price points, at least one of these Ethernet ports will be either 2.5 Gb/s or 5 Gb/s, which will drive adoption of higher speed cabling and equipment across the network. As Wi-Fi 6 technology matures to support greater than 5 Gb/s, two 5GBASE-T connections may be required to support a single Wi-Fi 6 WAP (this is often referred to as link aggregation) if 10GBASE-T uplink capacity is not supported by existing equipment (refer to figure 2, which depicts two horizontal link connections to each service outlet).
Link aggregation of two 10GBASE-T connections is foreseeable in the future as wireless capability continues to advance.
Although Wi-Fi 6 radio chips are equally or more efficient than prior generation wireless chips, they are doing significantly more complex signal processing and the amount of power required to energize Wi-Fi 6 devices is higher than for any previous implementation. In fact, due to complexity, both Wi-Fi 5 and Wi-Fi 6 WAPs are unable to work within the 13-watt budget of Type 1 Power over Ethernet (PoE) and must be supported by either a direct DC power adapter or 30-watt Type 2 PoE remote power. While safe for humans, Type 2 PoE remote power delivery, at an applied current of 600mA per pair, can produce up to 10°C (22°F) temperature rise in cable bundles7 and create electrical arcing that can damage connector contacts. Heat rise within bundles has the potential to cause bit errors because insertion loss is directly proportional to temperature. In extreme environments, temperature rise and contact arcing can cause irreversible damage to cable and connectors. Fortunately, the proper selection of network cabling, as described next, can eliminate these risks.
Existing wireless access devices, client devices and the back end network and cabling infrastructure may need to be up- graded in order to fully support Wi-Fi 5 and Wi-Fi 6 and Type 2 power delivery. In addition, operation in the 5 GHz transmission band (Wi-Fi 5 only operates in this band) requires relatively dense WAP coverage areas and existing prior generation (i.e., Wi-Fi 4) grid placement layouts may not be sufficient. For both new and existing wireless deployments, now is the time to seriously consider the wired cabling uplink infrastructure
Under all circumstances, the service outlets, patch panels, and other connecting hardware used in the channel should comply with IEC 60512-99-0018 to ensure that critical contact seating surfaces are not damaged when plugs and jacks are unmated under Wi-Fi 5 and Wi-Fi 6 remote powering current loads. In addition, the use of Siemon shielded category 6A and category 7A cables, which support longer channel lengths (i.e., less length de-rating is required at elevated temperatures to satisfy TIA and ISO/IEC insertion loss requirements) and are qualified for mechanical reliability up to 75°C (167°F), are recommended for Type 2 PoE remote powering applications in locations having an ambient temperature greater than 20°C (68°F). Furthermore, larger numbers of shielded cables may be bundled without concern for excessive heat build-up within the bundle.
Designing a cabling infrastructure to robustly support Wi-Fi 5 and Wi-Fi 6 deployment requires consideration of the switch, server, and device connection speeds commonly available today as well as strategies to support redundancy, equipment upgrades, and future wireless technologies. A grid-based class EA/category 6A zone cabling approach9 using service concentration points housed in zone enclosures is an ideal way to provide sufficient spare port density to support 2.5/5GBASE-T link aggregation at each WAP as necessary, while also allowing for more efficient port utilization when 10GBASE-T equipment connections become available. Zone cabling is highly flexible and enables rapid reconfiguration of coverage areas and conveniently provides additional capacity to accommodate next generation technology, which may require 10GBASE-T link aggregation. Additional WAPs can be easily incorporated into the wireless network to enhance coverage with minimal disruption when spare connection points in a zone cabling system are available.
Siemon recommends that each zone enclosure support a coverage radius of 13m (42 ft) with 24-port pre-cabled consolidation points available to facilitate plug and play device connectivity. For planning purposes, an initial spare port capacity of 50% (i.e., 12 ports unallocated) is recommended. Spare port availability may need to be increased and/or coverage radius decreased if the zone enclosure is also providing service to intelligent building devices and telecommunications outlets (TOs). Backbone cabling should be a minimum design of 25 Gb/s capable multimode optical fiber media to support Wi-Fi 5 and Wi-Fi 6 uplink capacity.
WAP deployments may be further simplified by utilizing a category 6A field-terminatable plug, such as Siemon’s
Z-PLUG®, at the equipment end of the installed channel. This approach eliminates the need to estimate the exact distance of cordage required, stock custom-length patch cords, source plenum cords, and address the problem of excessive cord tension or slack at the WAP. While field-terminatable plugs may be used in modular plug terminated link (MPTL) configurations, Siemon recommends minimum 2-connector channel topologies to facilitate adds, moves, and changes, field testing, and labeling.
What's in a name?
In the past, Wi-Fi access points, routers, and other devices were identified by letters and numbers, such as “802.11ac” or “a/b/g/n”, that corresponded to a specific IEEE 802.11 wireless Standard. In 2018, the Wi-Fi wAlliance re-branded and simplified Wi-Fi references as follows:- Wi-Fi 1: 802.11b (1999)
- Wi-Fi 2: 802.11a (1999)
- Wi-Fi 3: 802.11g (2003)
- Wi-Fi 4: 802.11n (2009)
- Wi-Fi 5: 802.11ac (2014)
- Wi-Fi 6: 802.11ax (2019)
Wi-Fi 6 delivers increased speed and capacity and more efficiently supports the increasing density of devices, while lowering latency and enhancing battery life. Growth for Wi-Fi 6 solutions will be explosive; with IHS Markit™ estimating IEEE 802.11ax-enabled device shipments to reach 193 million units in 20212. Specifying products that are part of the Wi-Fi Alliance® Wi-Fi CERTIFIED 6™ program is a recommended way to ensure that wireless products meet IEEE 802.11ax standards for interoperability and security.
Cabling Implications
Key cabling design strategies to ensure that structured cabling uplinks are ready to support the Wi-Fi 5 and Wi-Fi 6 wireless LAN devices addressed in this paper include:
- Specifying IEC 60512-99-001 compliant connecting hardware ensures that contact seating surfaces are not damaged when plugs and jacks are unmated under Wi-Fi 5 and Wi-Fi 6 remote powering current loads.
- Installing a minimum 25 Gb/s capable multimode optical fiber backbone to support increased Wi-Fi 5 and Wi-Fi 6 uplink capacity.
- Utilizing a grid-based zone cabling architecture to accommodate additional WAP deployments allows for rapid reconfiguration of coverage areas and provides redundant and future-proof connections.
- Using solid conductor cords, which exhibit better thermal stability and lower insertion loss than stranded conductor cords, for equipment connections in the ceiling or in plenum spaces where higher temperatures are likely to be encountered.
- Installing category 6A field-terminated plugs, such as Siemon’s Z-PLUG®, to eliminate common installation concerns associated with the use of pre-terminated cords at the equipment end of the installed channel.
- Providing two class EA/category 6A or higher performing horizontal cabling drops to each wireless access point (WAP) or router to facilitate link aggregation, which will be required by devices connecting into the Ethernet network with two ports or having greater than 5 Gb/s data rates.
Siemon’s shielded category 6A and category 7A cables are qualified for mechanical reliability up to 75°C (167°F), which enables support of the Type 2 PoE application over the entire operating temperature range of -20°C to 60°C (-4°F to 140°F).
Shielded systems are more thermally stable and support longer channel lengths when deployed in high temperature environments and larger number of shielded cables may be bundled without concern for excessive heat build-up within the bundle.
A Technology Evolution
Wi-Fi 6 has four times faster average throughput in dense deployment environments compared to Wi-Fi 5. This enhanced throughput is facilitated by an evolution of existing and proven Wi-Fi-5 communication algorithms. Like Wi-Fi 5, Wi-Fi 6 wireless transmission utilizes the techniques of beamforming to concentrate signals and transmitting over multiple send and receive antennas to improve communication and minimize interference (often referred to as multiple input, multiple output or MIMO). The signal associated with one transmit and one receive antenna is called a spatial stream and the ability to support multiple spatial streams is a feature of Wi-Fi 4, Wi-Fi 5, and Wi-Fi 6. Higher order modulation, an orthogonal frequency-division multiple access (OFDMA) signal scheme, which allows bandwidth to be divided according to the needs of the client, and synchronized uplink transmission are the key technology enablers that support faster Wi-Fi 6 transmission rates while ensuring backward compatibility with older Wi-Fi technology.
Quadrature amplitude modulation (QAM) is an analog and digital modulation scheme that is used extensively for digital telecommunications systems. Using this scheme, a four quadrant arrangement or “constellation” of symbol points is established with each point representing a short string of bits (e.g. 0’s or 1’s). Sinusoidal carrier waves that are phase shifted by 90 degrees are modulated using amplitude-shift keying (ASK) digital modulation or amplitude modulation (AM) analog modulation schemes and are used to transmit the constellation symbols. Figure 1 depicts a rudimentary example of a 16-QAM constellation for demonstration purposes. Note that there are four points in each quadrant of the 16-QAM constellation and each point equates to four information bits, ranging from 0000 to 1111. By comparison, the Wi-Fi 4 64-QAM scheme carries 6 bits of information per constellation point, the Wi-Fi 5 256 QAM scheme carries 8 bits of information per constellation point, and the Wi-Fi 6 1024-QAM carries an amazing 10 bits of information per constellation point.
Unlike Wi-Fi 5 devices that transmit exclusively in the 5 GHz spectrum, Wi-Fi 6 devices benefit from operation in both the 2.4 GHz and 5 GHz bands. This balances higher transmission rates achievable in the 5 GHz spectrum where there are more available non-overlapping radio channels, fewer devices operating, and less potential for interference with opportunities to transmit in the 2.4 GHz band where superior propagation characteristics (e.g., range and the ability to pass through building materials) may be better suited for IoT (Internet of Things) applications. Designing a flexible cabling infrastructure that can accommodate the addition of future WAPs and enable rapid reconfiguration of coverage areas can save headaches later. Figure 2 depicts a recommended zone cabling approach utilizing enclosures that house service concentration points (SCPs) with spare port capacity to facilitate connections to service outlets (SOs) that are positioned in a grid pattern. In addition, because most WAPs are located in the ceiling or in plenum spaces where higher temperatures are likely to be encountered, the use of solid conductor cords, which exhibit better thermal stability and lower insertion loss than stranded conductor cords3, are recommended for all equipment connections in high temperature environments. Refer to ANSI/BICSI 0074 and TIA TSB-162-A5 for additional design and installation guidelines describing a grid-based cabling approach that maximizes WAP placement and reconfiguration flexibility.
The Implications of Speed
Wi-Fi 5 and Wi-Fi 6 transmission channels that are 20 MHz wide are aggregated to create the “pipe” or “highway” for wireless transmission. Wi-Fi 6 technology allows radio transmission over either four or eight bonded 20 MHz channels supporting maximum throughput of 600.5 Mb/s and 1201 Mb/s, respectively. In addition, Wi-Fi 6 can accommodate up to eight antennas and their associated spatial streams for an unprecedented maximum theoretical data speed of 9.61 Gb/s! Note that, unlike full duplex balanced twisted-pair BASE-T Ethernet transmission where throughput is fixed in both the transmit and receive orientations, the speed specified for wireless applications represents the sum of upstream and downstream traffic combined. Figure 3 summarizes the key capability differences between Wi-Fi 4, Wi-Fi 5 and Wi-Fi 6 technologies.Because of the variables of channel bandwidth, number of spatial streams, and multi-user signaling mechanisms,
Wi-Fi 5 and Wi-Fi 6 implementations are highly configurable. In general, the lower end of the throughput range is targeted for small handheld devices with limited battery capacity such as smart phones, the middle of the throughput range is targeted towards laptops, and the highest end of the throughput range is targeted at specialized and outdoor applications where there is less device density compared with indoors. Figure 4 provides maximum theoretical speeds for currently available first and second wave Wi-Fi 5 and emerging Wi-Fi 6 implementations with their target end devices.
Possible future Wi-Fi 6 implementations are also shown, but these implementations may not be available for years, if at all. While this may seem surprising, consider that there are no 4-stream implementations of Wi-Fi 4 and manufacturers are bypassing 8-stream Wi-Fi 5 implementations in favor of Wi-Fi 6 products. Second wave Wi-Fi 6 devices will make use of multi-antenna beamforming, more efficient spatial utilization, and other strategies to improve throughput and long-range operation. The bottom line is that end-users can reasonably expect four times faster performance in dense environments and similar or slightly improved power consumption upon making the upgrade to Wi-Fi 6 gear.
When comparing Wi-Fi capabilities, it’s important to keep in mind that the maximum realizable data rate is impacted by the number of clients, protocol overhead, and the spatial distribution of end-user devices from the access point. In some cases, Wi-Fi 5 wireless data transfer rates are fast enough to saturate a 1000BASE-T copper balanced twisted-pair cabling link provided between the router and the server6. Proliferation of Wi-Fi 6 clients will certainly result in data rates regularly exceeding 1 Gb/s. 2.5GBASE-T and 5GBASE-T switches can reduce or eliminate the concern that a standard Gigabit Ethernet wired uplink ports will be a bottleneck to data transmission. However, it’s important to keep in mind that only class EA/category 6A and higher rated cabling is guaranteed to support 2.5/5GBASE-T over all installation environments and channel topologies up to 100 m.
The ability of Wi-Fi 6 to realize greater than 5 Gb/s wireless data rate capability has serious implications related to wired media selection for router to server and other uplink connections. Wi-Fi 6 access points connect into the Ethernet network with two ports rather than the traditional one port. Across all price points, at least one of these Ethernet ports will be either 2.5 Gb/s or 5 Gb/s, which will drive adoption of higher speed cabling and equipment across the network. As Wi-Fi 6 technology matures to support greater than 5 Gb/s, two 5GBASE-T connections may be required to support a single Wi-Fi 6 WAP (this is often referred to as link aggregation) if 10GBASE-T uplink capacity is not supported by existing equipment (refer to figure 2, which depicts two horizontal link connections to each service outlet).
Link aggregation of two 10GBASE-T connections is foreseeable in the future as wireless capability continues to advance.
Power Consumption
Although Wi-Fi 6 radio chips are equally or more efficient than prior generation wireless chips, they are doing significantly more complex signal processing and the amount of power required to energize Wi-Fi 6 devices is higher than for any previous implementation. In fact, due to complexity, both Wi-Fi 5 and Wi-Fi 6 WAPs are unable to work within the 13-watt budget of Type 1 Power over Ethernet (PoE) and must be supported by either a direct DC power adapter or 30-watt Type 2 PoE remote power. While safe for humans, Type 2 PoE remote power delivery, at an applied current of 600mA per pair, can produce up to 10°C (22°F) temperature rise in cable bundles7 and create electrical arcing that can damage connector contacts. Heat rise within bundles has the potential to cause bit errors because insertion loss is directly proportional to temperature. In extreme environments, temperature rise and contact arcing can cause irreversible damage to cable and connectors. Fortunately, the proper selection of network cabling, as described next, can eliminate these risks.
The Wired Infrastructure
Existing wireless access devices, client devices and the back end network and cabling infrastructure may need to be up- graded in order to fully support Wi-Fi 5 and Wi-Fi 6 and Type 2 power delivery. In addition, operation in the 5 GHz transmission band (Wi-Fi 5 only operates in this band) requires relatively dense WAP coverage areas and existing prior generation (i.e., Wi-Fi 4) grid placement layouts may not be sufficient. For both new and existing wireless deployments, now is the time to seriously consider the wired cabling uplink infrastructure
Under all circumstances, the service outlets, patch panels, and other connecting hardware used in the channel should comply with IEC 60512-99-0018 to ensure that critical contact seating surfaces are not damaged when plugs and jacks are unmated under Wi-Fi 5 and Wi-Fi 6 remote powering current loads. In addition, the use of Siemon shielded category 6A and category 7A cables, which support longer channel lengths (i.e., less length de-rating is required at elevated temperatures to satisfy TIA and ISO/IEC insertion loss requirements) and are qualified for mechanical reliability up to 75°C (167°F), are recommended for Type 2 PoE remote powering applications in locations having an ambient temperature greater than 20°C (68°F). Furthermore, larger numbers of shielded cables may be bundled without concern for excessive heat build-up within the bundle.
Designing a cabling infrastructure to robustly support Wi-Fi 5 and Wi-Fi 6 deployment requires consideration of the switch, server, and device connection speeds commonly available today as well as strategies to support redundancy, equipment upgrades, and future wireless technologies. A grid-based class EA/category 6A zone cabling approach9 using service concentration points housed in zone enclosures is an ideal way to provide sufficient spare port density to support 2.5/5GBASE-T link aggregation at each WAP as necessary, while also allowing for more efficient port utilization when 10GBASE-T equipment connections become available. Zone cabling is highly flexible and enables rapid reconfiguration of coverage areas and conveniently provides additional capacity to accommodate next generation technology, which may require 10GBASE-T link aggregation. Additional WAPs can be easily incorporated into the wireless network to enhance coverage with minimal disruption when spare connection points in a zone cabling system are available.
Siemon recommends that each zone enclosure support a coverage radius of 13m (42 ft) with 24-port pre-cabled consolidation points available to facilitate plug and play device connectivity. For planning purposes, an initial spare port capacity of 50% (i.e., 12 ports unallocated) is recommended. Spare port availability may need to be increased and/or coverage radius decreased if the zone enclosure is also providing service to intelligent building devices and telecommunications outlets (TOs). Backbone cabling should be a minimum design of 25 Gb/s capable multimode optical fiber media to support Wi-Fi 5 and Wi-Fi 6 uplink capacity.
WAP deployments may be further simplified by utilizing a category 6A field-terminatable plug, such as Siemon’s
Z-PLUG®, at the equipment end of the installed channel. This approach eliminates the need to estimate the exact distance of cordage required, stock custom-length patch cords, source plenum cords, and address the problem of excessive cord tension or slack at the WAP. While field-terminatable plugs may be used in modular plug terminated link (MPTL) configurations, Siemon recommends minimum 2-connector channel topologies to facilitate adds, moves, and changes, field testing, and labeling.
Conclusion
An evolution in technology forces consumers to stop and question legacy views about broadly deployed operating platforms or systems. Wi-Fi 6 is dually disruptive in that it requires both greater than 5 Gb/s capacity and Type 2 remote powering for optimum performance – swiftly making the wait-and-see stance concerning 10GBASE-T adoption in support of LAN applications a position of the past. A properly designed and deployed zone cabling architecture utilizing thermally stable shielded class EA/category 6A or higher cabling products engineered to withstand the maximum TIA and ISO/IEC ambient temperature of 60°C (140°F) plus the associated heat rise generated by 600mA Type 2 PoE current loads will ensure that your cabling infrastructure is an enabler for Wi-Fi 6 and future wave wireless applications
Related Products